A new kind of “phase transition” in water was first proposed 30 years ago in a study by researchers from Boston University. Because the transition has been predicted to occur at supercooled conditions, however, confirming its existence has been a challenge. That’s because, at these low temperatures, water really does not want to be a liquid, instead, it wants to rapidly become ice. Because of its hidden status, much is still unknown about this liquid-liquid phase transition, unlike everyday examples of phase transitions in water between a solid or vapor phase and a liquid phase.
This new evidence, published in Nature Physics, represents a significant step forward in confirming the idea of a liquid-liquid phase transition first proposed in 1992. Francesco Sciortino, now a professor at Sapienza Università di Roma, was a member of the original research team at Boston University and is also a co-author of this paper. The team has used computer simulations to help explain what features distinguish the two liquids at the microscopic level. They found that the water molecules in the high-density liquid form arrangements that are considered to be “topologically complex,” such as a trefoil knot (think of the molecules arranged in such a way that they resemble a pretzel) or a Hopf link (think of two links in a steel chain).
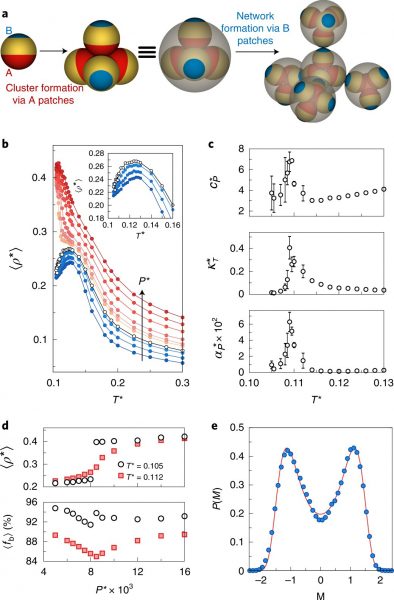
The molecules in the high-density liquid are thus said to be entangled. In contrast, the molecules in the low-density liquid mostly form simple rings, and hence the molecules in the low-density liquid are unentangled. Andreas Neophytou, a Ph.D. student at the University of Birmingham with Dr. Dwaipayan Chakrabarti, is the lead author of the paper. He said:
“This insight has provided us with a completely fresh take on what is now a 30-year-old research problem, and will hopefully be just the beginning.”
Colloidal water
The researchers used a colloidal model of water in their simulation, and then two widely used molecular models of water. Colloids are particles that can be a thousand times larger than a single water molecule. By virtue of their relatively bigger size, and hence slower movements, colloids are used to observe and understand physical phenomena that also occur at the much smaller atomic and molecular length scales. Dr. Chakrabarti, a co-author, said:
“This colloidal model of water provides a magnifying glass into molecular water, and enables us to unravel the secrets of water concerning the tale of two liquids.”
Professor Sciortino said:
“In this work, we propose, for the first time, a view of the liquid-liquid phase transition based on network entanglement ideas. I am sure this work will inspire novel theoretical modelling based on topological concepts.”
The team expects that the model they have devised will pave the way for new experiments that will validate the theory and extend the concept of “entangled” liquids to other liquids such as silicon. Pablo Debenedetti, a professor of chemical and biological engineering at Princeton University in the U.S. and a world-leading expert in this area of research, remarks:
“This beautiful computational work uncovers the topological basis underlying the existence of different liquid phases in the same network-forming substance. In so doing, it substantially enriches and deepens our understanding of a phenomenon that abundant experimental and computational evidence increasingly suggests is central to the physics of that most important of liquids: water.”
Christian Micheletti, a professor at the International School for Advanced Studies in Trieste, Italy, whose current research interest lies in understanding the impact of entanglement, especially knots and links, on the static, kinetics, and functionality of biopolymers, said:
“With this single paper, Neophytou et al. made several breakthroughs that will be consequential across diverse scientific areas. First, their elegant and experimentally amenable colloidal model for water opens entirely new perspectives for large-scale studies of liquids.
“Beyond this, they give very strong evidence that phase transitions that may be elusive to traditional analysis of the local structure of liquids are instead readily picked up by tracking the knots and links in the bond network of the liquid. The idea of searching for such intricacies in the somewhat abstract space of pathways running along transient molecular bonds is a very powerful one, and I expect it will be widely adopted to study complex molecular systems.”
Sciortino added:
“Water, one after the other, reveals its secrets! Dream how beautiful it would be if we could look inside the liquid and observe the dancing of the water molecules, the way they flicker, and the way they exchange partners, restructuring the hydrogen bond network. The realisation of the colloidal model for water we propose can make this dream come true.”
Provided by University of Birmingham [Note: Materials may be edited for content and length.]